Recent innovations in the field of genomic sequencing have given rise to new methods of sequencing genes (DNA/RNA). The newly developed techniques are categorized as Next Generation Sequencing (NGS).
The main advantage of NGS over other traditional methods, such as Sanger sequencing, is its ability to sequence several thousands of genes in a short time. NGS technology has been used in the healthcare industry for diagnosing patients, and mapping treatment plans and disease prognosis models1,5.
In this article, we cover the basics of NGS, the various technologies currently available in the market, some of their advantages and disadvantages, and clinical applications in the medical industry.
- How Does Next Generation Sequencing Work
- Techniques Used in Next Generation Sequencing
- Next Generation Sequencing Steps
- Sanger Sequencing vs Next Generation Sequencing
- Advantages of Next Generation Sequencing
- Next Generation Sequencing Technologies
- FAQs about Next Generation Sequencing
- Learn More About Next Generation Sequencing
How Does Next-Generation Sequencing Work?
The basic steps behind the NGS start from amplifying DNA/RNA strands, synthesizing and sequencing the new gene strands, and finally using all the newly generated genomic material to sequence the original input strand simultaneously across support systems.
Clinical applications of NGS
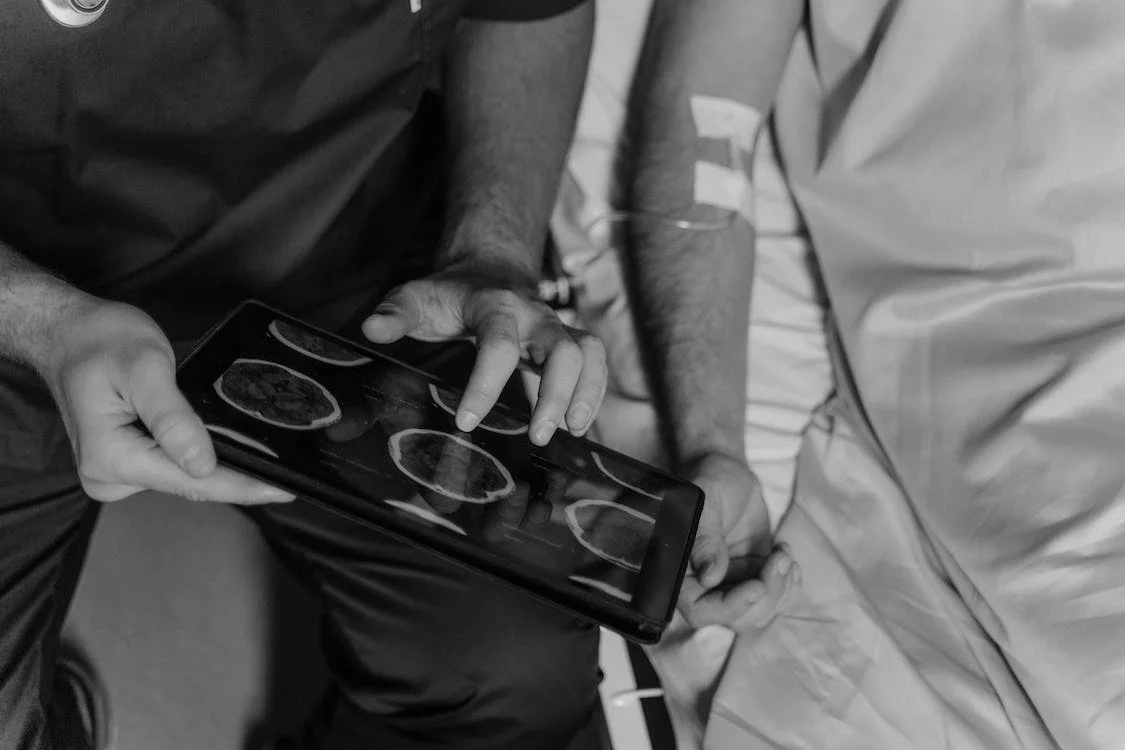
In clinical settings, NGS offers excellent precision and allows scientists and physicians to target different sequences of DNA all at the same time. For example, cancer is a multifactorial disease, and in genetics, multiple genes are implicated in its development.
For finding the mutations involved in cancer development, conventional techniques such as using molecular arrays require several assays to check for individual sites of mutations. With NGS, this process can be completed within one cycle, targeting all the mutations implicated in cancer.
One recent research with hematopoietic leukemia confirms that multiple gene mutations lead to cancer, and it is established that the interplay between mutations of three different genes is associated with subtypes of acute myeloid leukemia (AML).
Hence, as part of diagnostics, mutations at different locations are examined routinely in cancer patients. In addition, metastatic tumors can exhibit new changes in the genome other than the ones found in primary cancer1,2,5.
As a result, NGS has become an invaluable tool for medical and patient care professionals.
Techniques Used in Next-Generation Sequencing
New NGS methods of sequencing are termed next-generation techniques or second-generation techniques. “Second generation,” named such due to their superior capabilities and efficient performance compared to industry-standard techniques such as Sanger or the Maxam-Gilbert2.
The newly evolved next-generation techniques are grouped into two main categories: sequencing by hybridization and sequencing by synthesis (SBS).
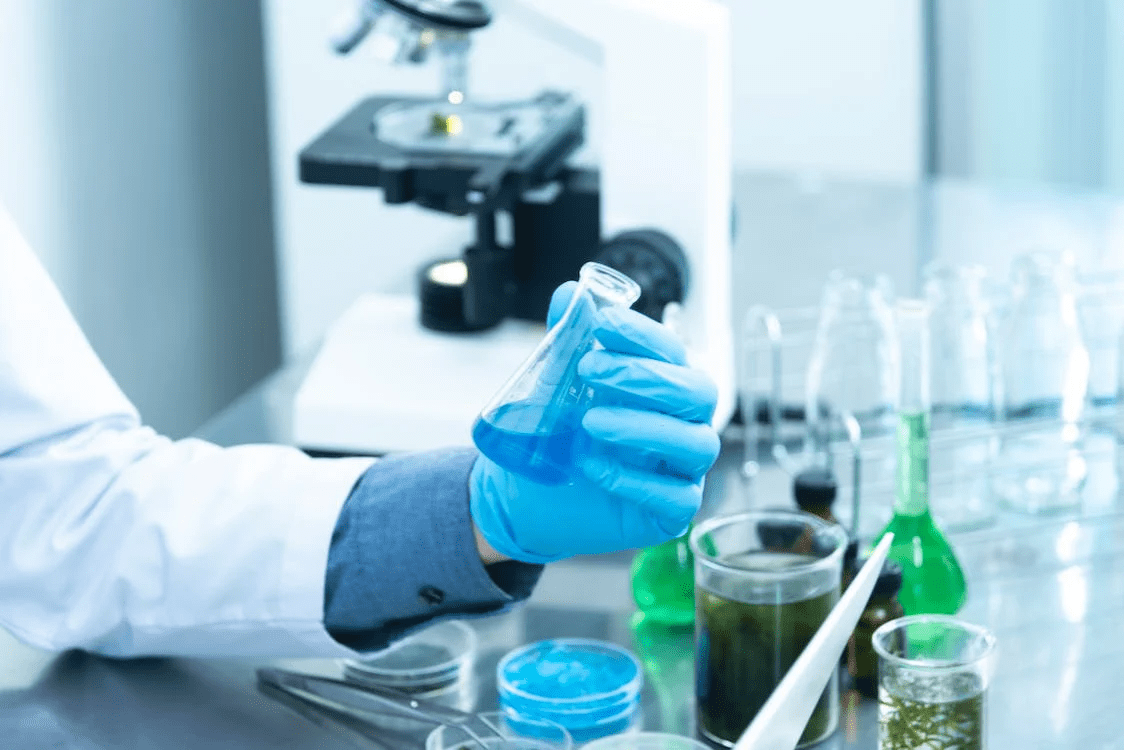
Sequencing by Hybridization
In this method, using arrays, single-stranded DNA (also known as probe) is allowed to pair with marked pieces of DNA of interest in a process known as hybridization. The DNA of interest is the target nucleic acid that is being sequenced.
In the end, the unpaired DNA fragments from the array are washed away; this process is repeated several times. Based on whether there was a pairing between the DNA of interest and the DNA probe, the gene sequence is determined.
Thus, using this data, scientists are able to form large gene sequences depending on pairing regions between the DNA probes and fragments to be sequenced.
Sequencing by Synthesis (SBS)
In this technology, there are several methods with a unique methodology. A general approach is to utilize a support system of tiny channels or chambers where the synthesis and sequencing can happen.
The DNA of interest (sample to be sequenced) is added to the thousands of chambers. These DNA fragments are amplified using PCR or DNA synthesis reactions.
Sequencing is done by detecting the precise reactions, where marked nucleotides are incorporated into the amplified DNA, which can be recorded in flow grams and reveal the nucleotide sequence of the DNA of interest.
Pyrosequencing
One of the earliest examples of NGS, although not widely in use. The technique used an innovative approach to detect nucleotide incorporation reactions. Pyrophosphate is a chemical that is released during the base (ATQC) incorporation process.
This method detects the presence of pyrophosphate to outline and sequence the DNA of interest. It comes under the category of SBS next-generation sequencing.
Ion semiconductor sequencing
The ion sequencing method converts nucleotide sequence information into digital data.
During the DNA synthesis reaction, pairing between nucleotides with their complementary base generates a hydrogen ion. The pH of the solution in which this reaction occurs is monitored. Therefore, any voltage change is detected by ion sensors.
If there is no pairing between bases, there is no change in voltage level. Voltage spikes are observed when there is nucleotide incorporation. By closely following the voltage spike patterns, the sequence is determined.
Again, Ion semiconductor sequencing is an SBS technique2.
Sequencing by ligation
This technique closely follows other SBS techniques. The difference is that sequence amplification is usually performed by DNA polymerases, but in this method, DNA ligase takes over this role.
Using a primer and a single-strand DNA, fluorescently labeled nucleotide probes are allowed to pair with their complementary sequences on the target DNA. The DNA ligase joins the primer with dye-marked probes forming a double-strand DNA.
After washing away the unpaired probes, fluorescence imaging can be used to identify the dye-marked probes and identify the DNA sequence.
Next Generation Sequencing Steps
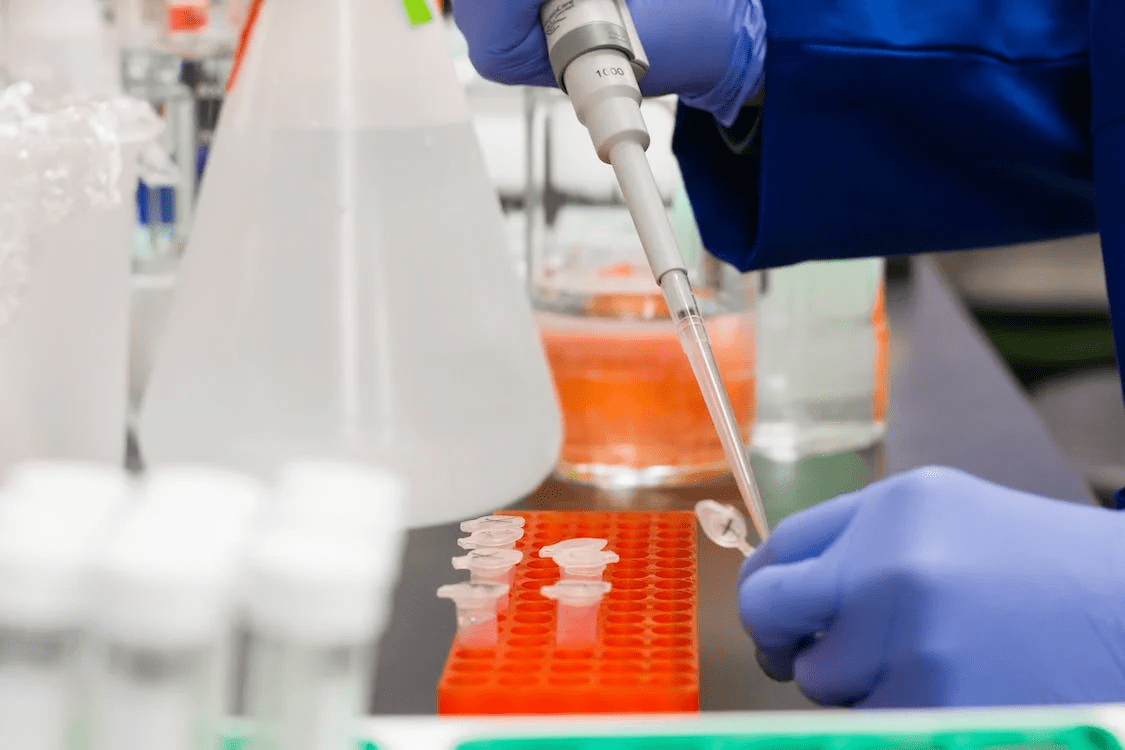
Due to the variety and differences in SBS techniques, here is a general overview of NGS steps for SBS techniques:
The workflow typically involves three basic steps: library preparation by fragmenting the DNA, amplifying and sequencing the fragmented DNA strand, and analyzing the output data.
Step 1: Library preparation
This first step is very important to the overall success of the NGS process. Here, genomic material is fragmented into single strands, and adapters are added to both ends for amplification.
Step 2: Sequencing
In the next step, the prepared libraries are loaded into support systems, fluorescently tagged, and modified DNA nucleotides start pairing with the single-stranded DNA in the libraries. The type of fluorescent signals detected by the sensors is used to identify the DNA sequence.
Step 3: Data analysis
In the final step, the fluorescent signals are identified using a software system and referred to as a base calling. This information is imported into analysis software, which can predict the accuracy of your entire NGS workflow.
Sanger Sequencing vs Next Generation Sequencing
What is Sanger sequencing
The Sanger sequencing method was under practice in 1977 and is still in use today. It uses modified nucleotides in the pairing process of complementary DNA. These nucleotides lack molecular components (OH group) required for amplification and are also fluorescently labeled.
As a result, DNA polymerases cannot extend the DNA strands. Therefore, resulting in the termination of the elongation process in that region.
The fluorescently labeled nucleotides can be detected using sequencing gels or software systems.
The difference between the two methods
The working principles behind Sanger sequencing and NGS are identical but not the same. NGS technologies are simply revised and improved versions of the Sanger method.
The main difference is that most NGS techniques do not rely upon modified nucleotides (dideoxy method) as reaction terminators.
Also, in NGS, the sequencing data is generated through exponentially more cycles of DNA amplification and synthesis processes, hence having a high throughput, and it can also be run in parallel with many samples, saving time.
Sanger Sequencing | Next Generation Sequencing | |
Advantages |
|
|
Limitations |
|
|
Advantages of Next-Generation Sequencing
Here are some main advantages of using NGS over Sanger.
NGS is capable of parallel sequencing
With NGS, multiple target regions can be analyzed and sequenced simultaneously. The ranges typically start from hundreds but can easily go over millions if needed and have higher sample sequencing volumes.
Offers higher sensitivity and bandwidth
When sequencing entire genomes, NGS techniques can detect errors such as deletions, insertions, and abnormalities across the whole genome.
NGS techniques are highly efficient
The input genomic materials for traditional methods such as Sangar are higher compared to NGS methods. In simpler terms, NGS is more efficient and can perform accurate sequencing with less input DNA.
Next Generation Sequencing Technologies
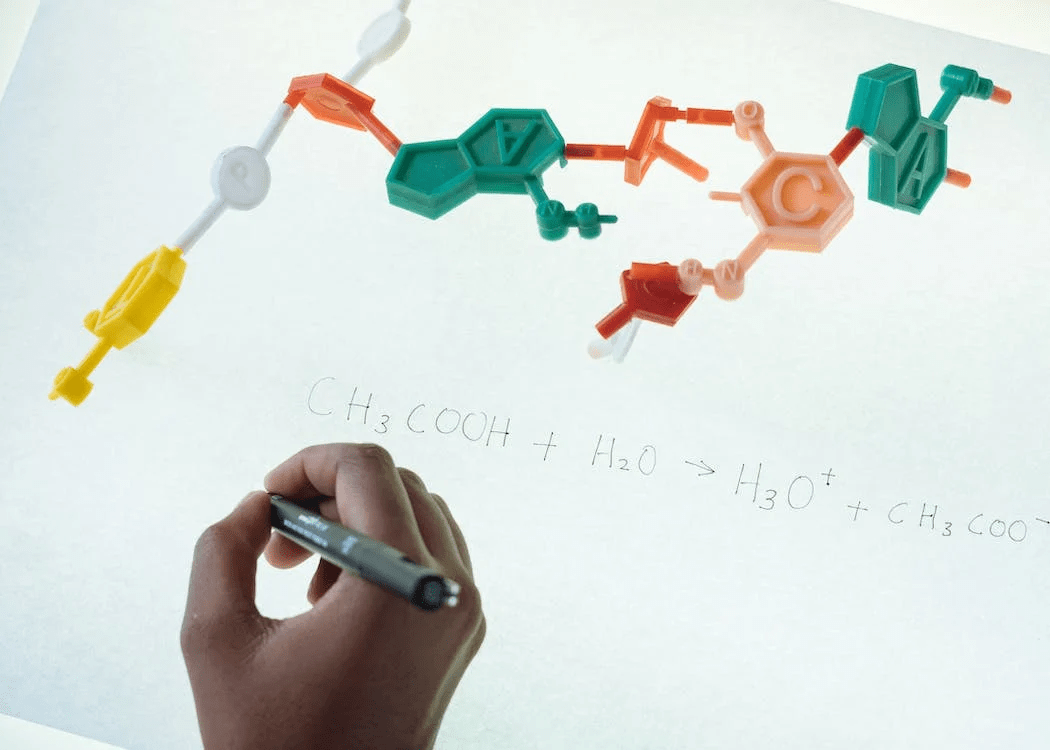
Here are some state-of-the-art NGS techniques that are currently available in the market:
Illumina (Solexa) sequencing
This technology is one of the best-known examples of sequencing techniques utilizing the SBS method. Sequencing is done by modifying and fluorescently labeling the four base nucleotides and incorporating them inside flow cells with thousands of samples.
In every workflow cycle, a labeled deoxynucleoside triphosphate is added during the DNA amplification process. This nucleoside serves as the terminator for the chain reaction.
Finally, the dye-labeled nucleotides are imaged. Detecting the paired base and each base is sequenced one at a time.
Roche 454 sequencing
It is similar to Ilumina technology in that sequencing is done by detecting optical signals as bases are incorporated into expanding DNA chains.
Roche 454 sequencing starts by fragmenting the input genomic material into smaller bits. It is unique in that instead of using flow cells — there are beads to which adaptors containing the input material can latch on.
Amplification of DNA or RNA strands begins after each sample is latched into one single bead. Beads are then washed away with four bases, one at a time.
The pairing between the bases releases light signals with which a graph is generated, and the sequence for the input material can be compiled using the software.
Thermo Fisher Ion Torrent: Proton / PGM sequencing
As we discussed above, the Thermo Fisher Ion Torrent does not rely upon optic signals for sequencing. Instead, it senses the pH changes in the support medium after adding deoxynucleoside triphosphate (dNTP, which releases hydrogen ions) to the gene amplification process.
DNA or RNA fragments are latched to beads using adapters and are amplified using PCR technology.
The washing process with dNTP is similar to Roche 454 sequencing, and pH changes are recorded and analyzed for sequencing the input fragments.
Guardant 360 CDx In Vitro System
Guardant 360 CDx is the latest NGS technology that has been approved by the FDA to detect gene mutations. It is a collection of multiple systems working together as a single unit to achieve high throughput sequencing and identify alterations such as single nucleotide variants, insertions, and deletions within the genome.
The process begins by extracting DNA from the patient’s blood. Gene sequencing is carried out using Illumina sequencing, and the obtained information is converted to digital data.
Analysis of digital data through the use of modern bioinformatics tools and customized pipelines tailored for disease profiles and mutations sets Guardant 360 apart from other NGS technologies6.
FAQs about Next Generation Sequencing
Who invented next-generation sequencing?
Various technologies evolved from the late nineties to what is termed as NGS today. The Roche 454 pyrosequencing, which uses the SBS techniques to sequence DNA, is the earliest commercially successful system for synthesis3.
It was developed by 454 Life Sciences in 2005, which was later acquired by Roche.
How much does next-generation sequencing cost?
The cost of NGS varies based on the platforms used for sequencing4. A general cost estimate in clinical applications would range from USD $555 to $5,169 for selected regions (exons). Whole genome sequencing can cost anywhere between USD $1,906 to $24,810.
Currently, for research purposes, there are kits available from various manufacturers; that advertise rates of USD 1,000 for sequencing the entire human genome.
Should I use next-generation sequencing or sanger sequencing?
It is best to go for NGS when there are more than 20 targets and sequencing involves higher sample volumes. It is cost-effective to go for Sanger if you are sequencing with less than 20 targets.
Learn More About Next Generation Sequencing
Interested in learning more about NGS?
Check our new publication on precision medicine and find out how the scope and applications of NGS can bring in changes in the healthcare industry, while discussing the need to ensure quality testing procedures and reproducible results with NGS technologies with renowned experts.
References
1. Qin D. Next-generation sequencing and its clinical application. Cancer biology & medicine. 2019;16(1):4–10.
2. Slatko BE, Gardner AF, Ausubel FM. Overview of Next-Generation Sequencing Technologies. Current Protocols in Molecular Biology [Internet]. 2018 Apr;122(1):e59. Available from: https://www.ncbi.nlm.nih.gov/pmc/articles/PMC6020069/
3. Kulski JK. Next-Generation Sequencing — An Overview of the History, Tools, and “Omic” Applications. Next Generation Sequencing – Advances, Applications and Challenges [Internet]. 2016 Jan 14; Available from: https://www.intechopen.com/books/next-generation-sequencing-advances-applications-and-challenges/next-generation-sequencing-an-overview-of-the-history-tools-and-omic-applications
4. Schwarze K, Buchanan J, Fermont JM, Dreau H, Tilley MW, Taylor JM, et al. The complete costs of genome sequencing: a microcosting study in cancer and rare diseases from a single center in the United Kingdom. Genetics in Medicine [Internet]. 2019 Jul 30;22(1). Available from: https://www.nature.com/articles/s41436-019-0618-7
5. Recognizing the value of next-generation sequencing and call-to-action for its quality assurance and standardization [Internet]. [cited 2022 Dec 17]. Available from: https://apacmed.org/content/uploads/2022/10/The-value-of-quality-next-generation-sequencing-in-the-APAC-region_06C.pdf
6. Lanman RB, Mortimer SA, Zill OA, Sebisanovic D, Lopez R, Blau S, et al. Analytical and Clinical Validation of a Digital Sequencing Panel for Quantitative, Highly Accurate Evaluation of Cell-Free Circulating Tumor DNA. Hoheisel JD, editor. PLOS ONE. 2015 Oct 16;10(10):e0140712.